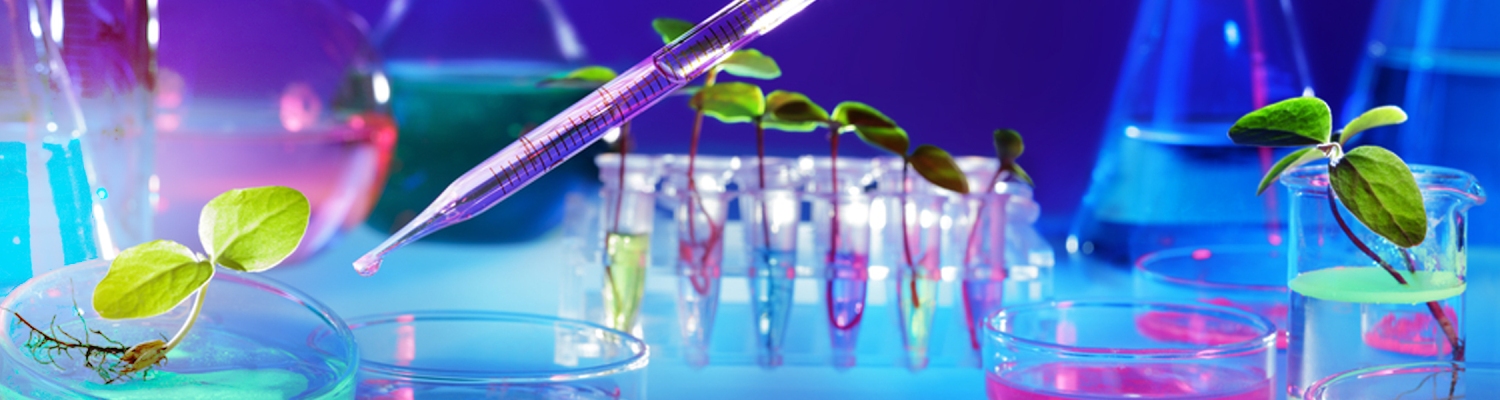
Ramona Massoud1 and Alaleh Zoghi2*
Received:February 02, 2023; Published:February 09, 2023
*Corresponding author: Alaleh Zoghi, Department of Food Science and Technology, National Nutrition and Food Technology Research Institute, Faculty of Nutrition Science and Food Technology, Shahid Beheshti University of Medical Sciences, Tehran, Iran
DOI: 10.32474/CTBM.2023.03.000163
Contaminants such as mycotoxins, heavy metals, and chemicals in food are one of the major concerns of our world nowadays. So, food decontamination with the help of microbial biomass is a cheap, easy, efficient, and green method known as bio removal. Bifidobacterial are able to reduce the availability of contaminants in food products. The purpose of this review is to summarize the Bifidobacterium species’ interesting role in food bio-decontamination. After a brief glance at the definition of bifidobacterial strains, their effect on human health is described. After that, food contaminants, including mycotoxins, heavy metals, and chemicals are defined and the bio-decontamination activity of bifidobacterial genera is described. Additionally, the effect of some factors in bio removal efficiency and the bio removal mechanism are explained. It is worth mentioning that this review article can be exerted in different food and beverage industries to eliminate the contaminants in food products.
Keywords:Probiotics; bio removal; bioremediation; mycotoxin; heavy metal
Food plays an important role in human beings’ health and energy and seems vital for growth, body organs, tissues, metabolism, and functions. Along with nutrients, food may contain toxins (naturally produced during the processing and storage of food), mostly in low levels and not in adverse effects levels [1]. Food and water are being contaminated by industrial activities increasingly and they affect human and food safety [2]. Food and water contaminants would cause ecological unbalance. Therefore, decontamination is necessary to reduce the contaminants of foodstuffs to guarantee their safety. The current approaches, the physical and chemical degradations, are really costly and time-consuming along with unfavorable nutritional loss. Bioremoval is an economically effective method for removing and reducing contaminants in foodstuffs. Different microorganisms are used to detoxify and deteriorate hazardous pollutants in food products [3,4]. It has already been proved that bifidobacterial is an effective alternative in bio-decontamination. Some studies have reported the bioremoval of contaminants such as mycotoxins (patulin (PAT), aflatoxins (AF), zearalenone (ZEA), ochratoxin A (OTA), and microcystin-LR), heavy metals (lead (Pb), cadmium (Cd), and arsenic (As)), and chemical substances (bisphenol A and benzo(a)pyrene) by bifidobacterial [5-9]. Bifidobacteria are able to decontaminate foodstuffs via various mechanisms like using viable or dead microorganisms or their specific produced enzymes [10]. The nonviable bacteria’s ability to detoxify is significant as their viability would decrease through passing the digesting system. Contaminant absorption by the bifidobacterial cell wall is a unique mechanism. Generally, this article reviews the role of Bifidobacterium (B.) strains in the reduction and inactivation of different contaminants in food products.
Data collection, inclusion and exclusion criteria, and bibliographic research were all completed. Following a thorough search of the following databases, pertinent published studies were chosen: PubMed, Scopus, Science Direct, ISI web of knowledge, and Google Scholar; using keywords like “Mycotoxin”, “heavy metal”, “Reduction”, “Bifidobacterium”, “chemical contaminants”, “bio-decontamination”, “Food”, “degradation”, and “biological binding”. In order to choose some more publications, the reference lists of the relevant scientific articles were carefully examined. The selected literature was categorized according to the reduction of contaminants in foodstuff using different strains of bifidobacterial and was reviewed independently in full text.
Bifidobacterium is a Gram (+), non-spore and non-motile, anaerobic bacteria that generate acetic and lactic acids. The optimum growing temperature and pH are around 36 - 38°C and 6.5 to 7 respectively. Bifidobacterium can synthesize riboflavin and thiamin [11]. The bifidobacteria have been assigned to some genera like Lactobacillus, Bacillus, Bacteroides, Nocardia, Actynomices, Bacterium, Actinobacterium, Corynebacterium and Bifidobacterium. Some species are based form bifidus, which means divided in Latin [12]. Also, they are the inhabitant of the gastrointestinal tract and some are known as probiotics [13]. “Probiotics are viable microorganisms which cause some health benefits in sufficient quantities” described FAO [14]. Lactose intolerance reduction, improving the immune system, cholesterol-lowering effect, diarrhea inhibition, cancer prevention, and antimutagenic and anticarcinogenic impacts are some of the mentioned health benefits [15]. It is reported that B. lactis HN019 is able to improve immunity. Also, Bifidobacterium can boost hydrogen peroxide, nitric oxide, and interleukin-6 production [16]. The natural microflora in our gastrointestinal tract is wide-open to some genotoxic combinations. Some gut bacteria are able to bind mutagenic pyro lysates to reduce the mutagenicity efficiently.
Bifidobacteria are one of the most important bacteria in the gut. They are also consumed as dietary supplements [17]. The bifidobacteria’s cell wall has peptidoglycans, lipoteichoic and teichoic acids, neutral polysaccharides, and a proteinaceous layer (S). The components pose important functions like binding to macromolecules like toxins [18]. Peptidoglycan includes disaccharide muramic acid connected by pentapeptides. Some Bifidobacterium generates exopolysaccharides including glucose, galactose, rhamnose, mannose, N-acetylglucosamine, and N-acetylgalactosamine. These matrices preserve microorganisms by linking to the epithelial cells along with improving the immune system. It was proved that some bacteria could adsorb lead and mercury as containing exopolysaccharides [19]. The bifidobacteria introduced a desirable relationship with our gastrointestinal health and nutrition. Nowadays bifidobacteria have gained attention for probiotic function and not only for human consumption but also for animals.
Mycotoxins
Mycotoxins are one of the major concerns as they pose toxicity, carcinogenic, mutagenic, and different side effects. Patulin, aflatoxins, ochratoxins, Zearalenone, fumonisins, cyanotoxins, and trichothecenes are among the most hazardous food toxins [20]. Aspergillus (A.) species are able to generate aflatoxins (AFs), the carcinogenic hazardous metabolites. AFB1 and AFM1 are the most common AFs in food products. A reduction in amylase, lipase, and chymotrypsin activity in presence of AFs was reported [21,22]. A. ochraceus and carbonarius, and Penicillium verrucosum are the main producers of OTA. Ochratoxins are hazardous food contaminants, heat stable, and related to tumors promotion in humans and animals. OTAs contaminants are reported in cereals, dried fruits, cocoa, coffee, wine, and spices. Patulin is water-soluble and generated from Aspergillus and Penicillium species. It was observed in apples, peaches, grapes, and blueberries. PAT would result in ulceration, RNA and DNA inhibition, stomach irritation, gastrointestinal problems, lung congestion, and vomiting [23,24]. ZEA is a Fusarium fungi mycotoxin metabolic product commonly found in cereal crops. This toxin hurts the kidneys and liver and decreases the immune system. Cyanotoxins are generated by Cyanobacteria and are mainly considered as water contaminants. Microcystin-LR is one of the common cyanotoxins including leucine and arginine. It is reported to be a tumor promoter by preventing protein phosphatases 1 [25].
Heavy Metals
Some industrial activities, chemicals, effluents, pesticides, and mining have been polluting our environment, water, and food continuously [26,27]. Heavy metals are one of the most hazardous elements as they are non-biodegradable, and their accumulation poses toxic effects on the human body. Lead, cadmium, and arsenic are among the hazardous toxic metals. It was reported that cadmium exposure results in renal cancer, kidney damage, and osteoporosis; lead is accumulated in bones and teeth and may develop anemia [28,29].
Chemical Contaminants
Chemical contamination adversely affected foodstuffs and would produce a group of chemical combinations. The sources of the contamination can be natural from volcanic activity, rock weathering, and earthquakes or anthropogenic like using antibiotics, pesticides, and fertilizers [30]. Bisphenol A is produced from epoxy resins and polycarbonate plastics. Bisphenol A leaching into beverage and food products from packaging has been reported [31]. Bisphenol A poses an endocrine system and would result in diabetes, obesity, fertility problems, and some cancers [32]. Also, food safety relates to some cancerogenic compounds such as polycyclic aromatic hydrocarbon benzopyrene. It is present in cereals, grilled meat, seafood, smoked fish, oil, fruits, and vegetables. Benzopyrene is able to disperse in the bladder, kidneys, and liver and cause oxidative stress and make trouble in cell signaling [33].
The role of ?Bifidobacteria in the reduction of mycotoxins was reported in some research. OTA (22%) and PAT (82%) biodetoxification by B. animalis were stated. It is reported that B. longum was able to remove OTA more than PAT [34]. Hatab et al. [35] compared PAT removal by B. bifidum and animalis at 37°C in 24 h. The highest removal was with unviable bacteria; B. Bifidum (58%) and then B. animalis (25%). They also reported the ability of Lactobacillus rhamnosus and helveticus, B. bifidum, and animalis for PAT bioremoval in apple juice at 37°C, pH 4 after 24 h. Results represented 82 % of PAT reduction in the initial toxin concentration of 100 μg/ mL at 30°C by L. rhamnosus [36]. The reduction of microcystin-LR (59%) by B. lactis Bb12 after 24 h at 37°C was stated [37]. According to this research, higher bioremoval was observed in higher temperatures, pH, and bacterial concentration. AF bioremoval by some Lactobacillus spp and B. bifidum in skim milk was evaluated [38]. The removal relates to the strain of bacteria and B. bifidum removal was the highest (45%). AFs bioremoval from aqueous solution was reported by B. breve, L. rhamnosus, and Propionibacterium shermanii [39]. The highest AF elimination (21.4%) was observed by B. breve. The binding ability of AF by B. bifidum and B. animalis in fermented milk was reported by Martínez et al. [40]. AFM1 removal (100%) in UHT skim milk with B. lactis was stated and binding to the toxin’s available sites was described as the bioremoval mechanism [41]. Abdelmotilib et al. [42] reported that using the bacterial species mixture (L. acidophilus, L. plantarum, and B. bifidum) resulted in the highest AFM1 removal (88 %). Mohammadi et al. [43] stated B. bifidum (107 CFU/ml) ability to eliminate AFM1 in Feta cheese.
B. longum was reported to remove lead and Cadmium by binding to the teichoic acids and polysaccharides of the cell wall [44]. Xing et al. [45] represented lead elimination in laying hen’s gut content by B. longum through binding to the bacterial surface. Oishi et al. [46] reported the ability of B. breve to enhance the excretion of oral bisphenol A in rats. The bacteria reduced the amount of bisphenol in the blood and raised bisphenol excretion in the feces of rats. Bisphenol A seems two times higher in the sediment fraction of excrements. According to this research, heat-killed bacteria represented a higher bisphenol A secretion than viable cells. Also, the results showed that bisphenol A binding by bifidobacterial was the primary way of detoxification compared to enzymatic mechanisms [47]. B. bifidum and L. reuteri are able to remove benzopyrene through binding at rates of 96% and 97% respectively [48]. It is mentioned that the complicated structure of peptidoglycans in Bifidobacterium is significant in the binding mechanism of benzopyrene. Peptidoglycans in B. infantis represent the highest binding benzopyrene [49]. The results confirm the role of the cell wall in benzopyrene binding and highlight the physical binding related to the cell wall compound in the bioremoval process.
The type and quantity of contaminant, inoculum size, temperature, and bifidobacterial spp. Are mentioned as the important factors in the bioremoval process. It has been reported that the ability of Bifidobacterium for bio-decontamination relates to pH value. There was a reduction in PAT binding by B. bifidum and B. animalis in less acidic media. The incubation time is the other significant factor affecting bio-decontamination. A 90% of ZEA removal by Lactococcus lactis was observed during 20 min of incubation, whereas ZEA removal was reported by Bifidobacterium spp. and the bioremoval enhanced and reached 88% after 12 h of incubation [50]. Also, it is stated that B. bifidum was able to bind 23% of AFM1 at the start of the trial rapidly. Bifidobacterium pretreating affects the biosorption process. The pretreating results in protein denaturation, changes in charge distribution, and the hydrophobic surface of bacteria that develops the absorption [51]. Bioremoval is enhanced due to the changes in the cell surface after heat treatment and absorption facilitated. The pretreating of bacteria by acid reduced the thickness of the cell wall as the monomers left proteins and the linkage of glycose and amide in peptides and polysaccharides hackled [52]. Alkaline pretreating reduces the covering compounds of the surface and therefore the binding sites availability changes, the acidic groups neutralize, and the electronegativity of the cell surface changes [53]. Nybom et al. explained the relevance of proteolytic activity in B. lactis and microcystin elimination based on glucose as the main energy resource. The bio-decontamination progresses by temperature rising, bacteria amount, and alkaline condition but after heat, acid, and ethanol treatments the bioremoval reduces.
Bifidobacterium spp. Assist in bio-decontaminants of foodstuffs via specified mechanisms. They are able to produce proteolytic enzymes, the proteinase of cell-wall that hydrolyze proteins. The peptide transporters transport the peptides inside the cells and the inter peptidases cut them into various amino acids. Another mechanism was explained for biosorption with the help of the bifidobacterial cell wall; the interaction of bacteria’s metabolites (bioactive peptides, phenolic compounds, and fatty acids) and the contaminants. The reaction of the contaminants and the bacteria’s metabolites leads to forming some nontoxic forms in food products. Bifidobacteria can make non-covalent bonds between the cell surfaces and the contaminants [54]. different research reported the variety of binding bacterial spp. And pollutants as there are various cell wall structures. It has been shown that the two main components responsible for the binding are cell wall polysaccharides and peptidoglycan. In general, it is stated that physical adsorption is more important in bio-decontamination than chemicals. The bio absorption of heavy metals binding with exopolysaccharides is described by a metallic ions interaction with the functional groups of exopolysaccharides (carboxyl, hydroxyl, acetate, sulfate, phosphate, and amine groups). It was stated that metal biosorption is a physicochemical process with ion exchange, precipitation, and complexion [55]. Using Bifidobacterium for bio-contaminants in foods relates to the stability of their complex in our gastrointestinal tract. Bifidobacteria can cohere to the intestine, and they release after binding to contaminants [56]. Releasing contaminants into solutions is influenced by various factors such as cell wall structure, inoculum size, temperature, pH, and type and level of contaminant [57-70]. According to all the above, it is worth mentioning that the in vitro experiments have to be tested in vivo situations as contaminants may take apart from the bacteria after consumption and enter our body and have some negative health effects.
Through tainted food, humans are exposed to a wide range of substances, including environmental contaminants. Through the alteration of the gut microbial balance, often known as dysbiosis, long-term exposure to these pollutants is linked to a number of intestinal illnesses. An important topic of research right now is how to lessen the harmful effects of food pollutants and get them out of the host body. Green technology to reduce numerous health concerns is food bio-decontaminant. It has been demonstrated that some microorganisms, such as bifidobacteria, are capable of removing contaminants from food. Table 1 shows some of the recent research in which Bifidobacterium strains have been used for the bio-decontamination process in foodstuffs. The advantages of employing Bifidobacterium strains for bio-decontamination include direct food application, straightforward bioactivity, economy, low cost, and speed. Different incubation times, temperatures, pH levels, inoculum sizes, and pollutant concentrations affect how well they can bio-decontaminate. More research is required to identify the precise bio-decontamination mechanism as well as the ideal circumstances for boosting process yield. Despite considerable studies on Bifidobacterium spp. use in food bio-decontamination, additional information on the useful advantages in the food business is still required. It is important to note that in order to determine the impact of the Bifidobacterium spp. on the availability and toxicity of pollutants, all in vitro results should be protected by in vivo experiments.
Table 1. Recent research regarding Bifidobacterium strains applications for different contaminants bio removal in foodstuffs.
Abbreviations: Lactobacillus: L.; Saccharomyces: S.; Bifidobacterium: B.; Lactococcus: Lc.; Lead: Pb; Cadmium: Cd.
No conflict of interest was declared.
Bio chemistry
University of Texas Medical Branch, USADepartment of Criminal Justice
Liberty University, USADepartment of Psychiatry
University of Kentucky, USADepartment of Medicine
Gally International Biomedical Research & Consulting LLC, USADepartment of Urbanisation and Agricultural
Montreal university, USAOral & Maxillofacial Pathology
New York University, USAGastroenterology and Hepatology
University of Alabama, UKDepartment of Medicine
Universities of Bradford, UKOncology
Circulogene Theranostics, EnglandRadiation Chemistry
National University of Mexico, USAAnalytical Chemistry
Wentworth Institute of Technology, USAMinimally Invasive Surgery
Mercer University school of Medicine, USAPediatric Dentistry
University of Athens , GreeceThe annual scholar awards from Lupine Publishers honor a selected number Read More...